Redox Flow Batteries – Large Energy Storage Systems of the Future?
Interview of November 6, 2020
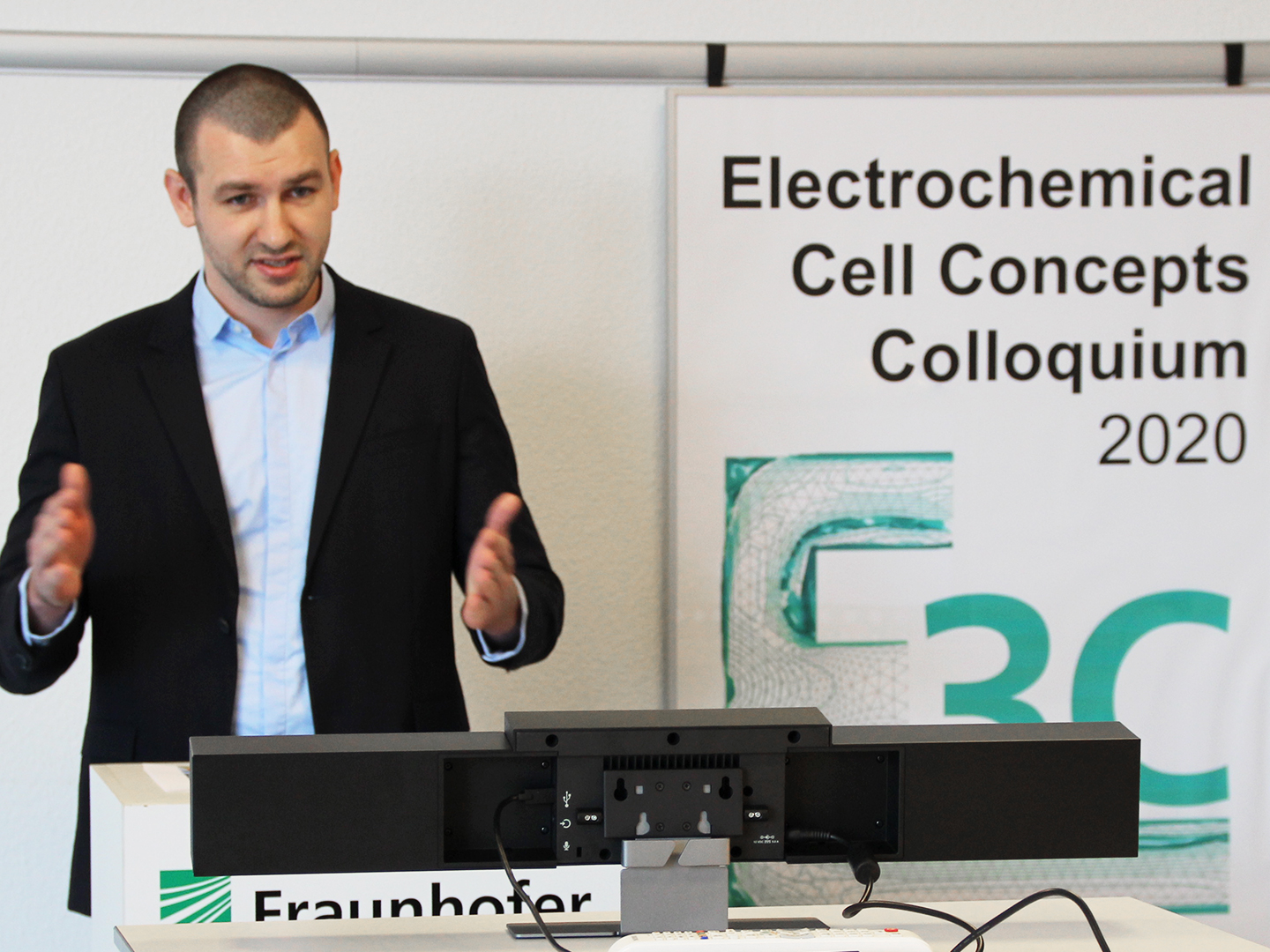
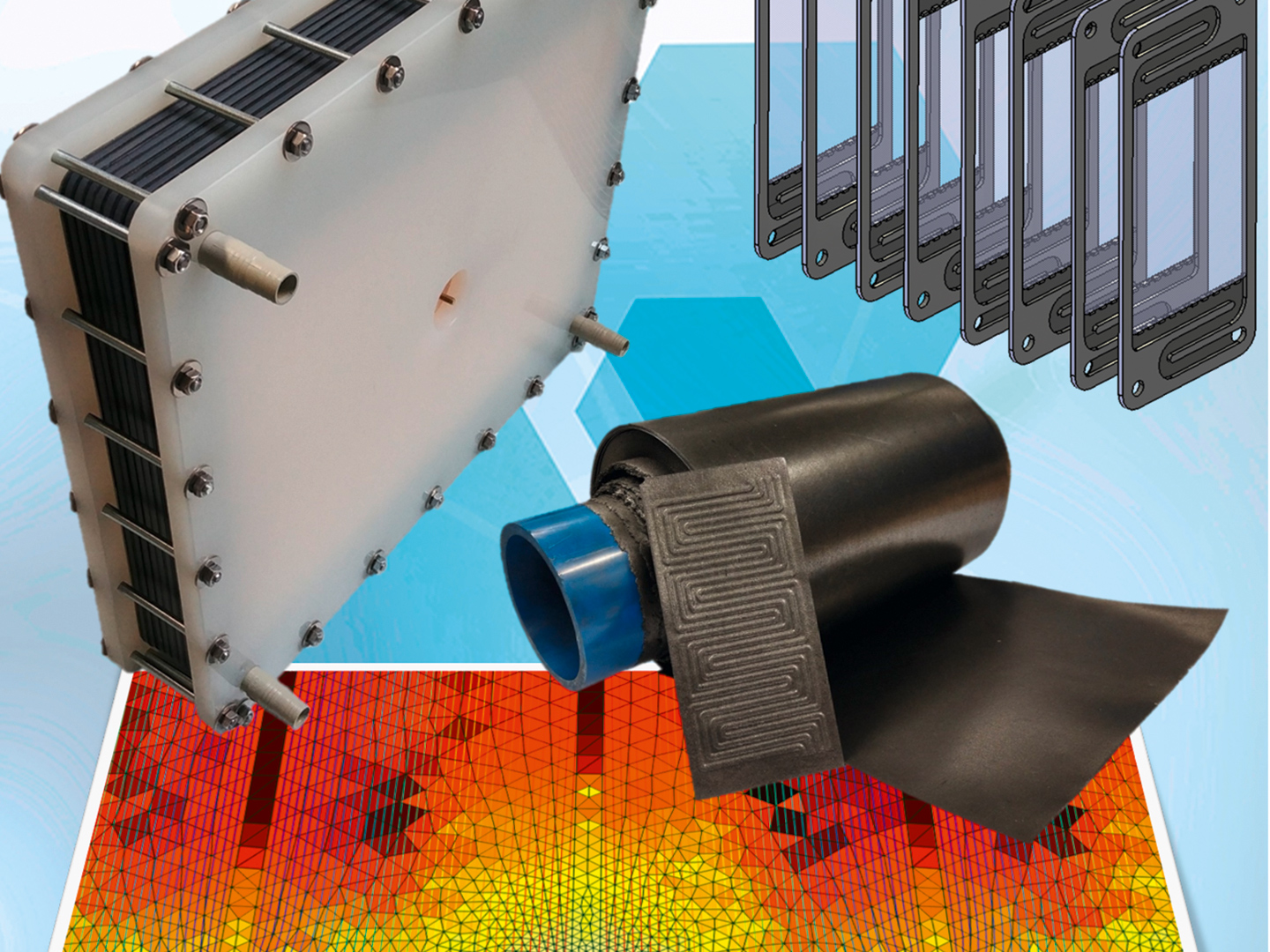
While the power and storage capacity of conventional non-flow batteries such as lead-acid or lithium-ion batteries are in a fixed ratio to each other, they can be scaled in redox flow batteries. Due to their very long calendrical operating life with theoretically unlimited cycle stability, a very low self-discharge rate and above all a particularly high level of operational safety, redox flow batteries are predestined for the reliable stationary storage of small and large amounts of energy. In contrast to other battery technologies that address comparable areas of application, redox flow batteries are not flammable and cannot explode. A current research focus is also on the development of alternative cell chemistries, so that the use of environmentally neutral storage media is also foreseeable in the future. Jan Girschik, scientist in the field of energy at Fraunhofer UMSICHT, explains functions and applications.
What are the main differences between redox flow and non-flow batteries such as lithium-ion or lead-acid batteries?
Jan Girschik: Unlike lithium-ion and lead-acid batteries, redox flow batteries are external energy storage systems. This means that the actual storage medium is stored outside the battery's energy conversion unit. Two liquid electrolyte solutions act as the storage medium, which are stored in simple external tanks and are only pumped through the actual battery cells for charging and discharging. In the battery cells, the electrolytes usually flow through and over porous graphite felt electrodes, where the conversion from electrical to chemical energy takes place during charging and from chemical to electrical energy during discharging.
The electrodes in redox flow batteries therefore have a purely catalytic effect, which also prevents degradation. And this results in a theoretically unlimited cycle stability and long operating life. The separation of the energy conversion and energy storage unit is a major advantage of flow batteries compared to non-flow systems, because it allows the independently and flexible scalability of the power output and storage capacity and furthermore the subsequent adjustment of these parameters. As in non-flow batteries, the performance can be increased by a scale-up, i.e. by increasing the active cell areas, or by a numbering-up, with a larger amount of battery cells. While a scale-up primarily generates technological challenges such as tightness at high internal pressures, a numbering-up always means additional electrical and hydraulic losses as well as higher production and material costs. However, to increase the storage capacity of flow batteries, only the amount of electrolyte used needs to be increased. This means that larger tanks can simply be used or the existing tanks can be supplemented with additional ones. This flexible scalability of the two parameters enables the redox flow battery to be dimensioned precisely for the respective storage task.
What storage tasks do you see as the application scenario for redox flow batteries?
Jan Girschik: Basically, redox flow batteries are particularly suitable for stationary energy storage tasks, but they are also very flexible in their use. The main area of application in the private sector is the intermediate storage of solar and wind energy to increase the use of the own electricity and thus the degree of self-sufficiency. In the commercial sector, redox flow batteries can be used by grid operators, for example, for load shifting and peak shaving to relieve the load on the power grids. They can be used to provide control energy as well as buffer storage at EV charging stations and, due to the very low, almost non-existent self-discharge, also as emergency power storage. The electrolytes can only react when they are in the battery cells. When the flow battery is not in operation and the pumps are switched off, self-discharge only takes place between the insignificantly small amounts of electrolyte remaining in the energy conversion unit. The external electrolyte tanks can therefore theoretically be placed in a cabinet, closed, and taken out again with the same state of charge when they are needed.
Do you think redox flow batteries could be introduced worldwide and what are the potential obstacles?
Jan Girschik: A worldwide use as a complementary technology is quite conceivable. The redox flow battery is already being researched and successfully used in many countries. In Dalian, China, for example, the world's largest vanadium redox flow battery with a final power output of 200 MW and a storage capacity of 800 MWh is being built. The vanadium flow battery is currently the most common used type, as the vanadium electrolytes have a good potential range and can be regenerated repeatedly. However, the criticality and price development of the basic materials of the electrolyte can be potential obstacles for a broad market penetration. The same applies to the currently higher procurement costs of battery cells including peripherals compared to other electrochemical storage systems, also because there is no mass production of redox flow batteries yet.
These obstacles could be overcome, for example, by increasing the resource efficiency by using innovative materials and optimized cell designs. In addition, production could be increasingly automated and supply chains could be established. Another important point is the use of alternative, cheaper and available cell chemistries such as in hybrid flow batteries or organic redox flow batteries.
What research topic on the redox flow battery are you currently working on?
Jan Girschik: In the area of flow batteries, we are currently focusing on the development of strategies for efficient flow management in battery cells and stacks. The generic term efficiency includes system efficiency on the one hand and resource efficiency on the other, and both are mutually dependent. As already mentioned, to increase the performance of a flow battery, a scale-up can reduce material and production costs compared to a numbering-up. Additionally also the impact of efficiency losses, for example due to shunt currents, can be reduced. However, large active areas in the battery cells also mean that the electrolytes have to be pumped over a greater distance through the porous electrodes, which is associated with considerable pressure losses - especially with higher and highly viscous electrolytes. In addition, potential differences can occur in the electrode, since the electrolytes have a different state of charge when they leave the battery cell than when they enter. In order to be able to generate an even and efficient fluid flow through large-scale flow battery cells with low pressure losses, we have developed and patented a number of solutions which we are currently optimizing and further developing.